Crane Collapses: Why It Happened And How To Avoid
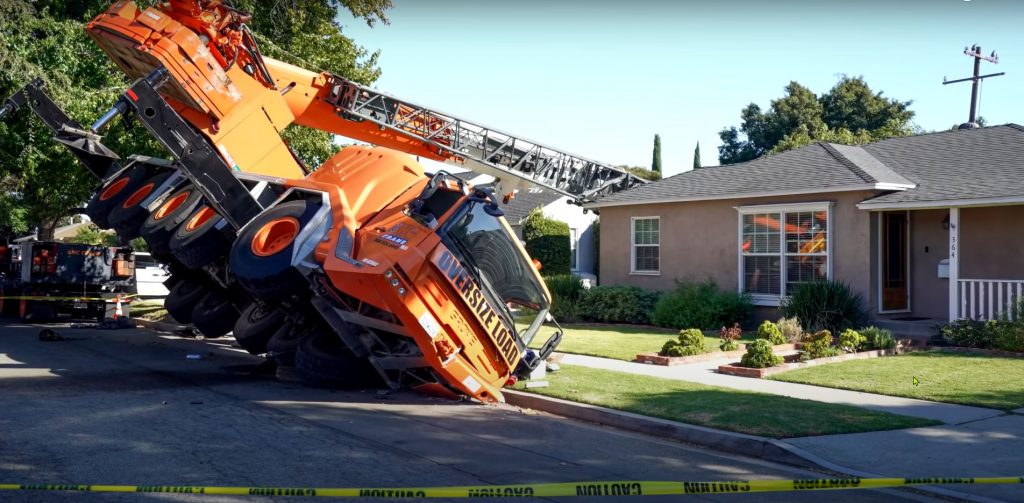
Cranes are dangerous.
Anytime something goes up, there’s a chance it might fall down. Keep that in mind next time you climb a ladder. But lifting stuff up and getting it back down safely is pretty much a Crane’s only job. So why do so many of them fall down? Let’s walk through some of the biggest crane disasters in modern history to try and understand. I’m Grady, and this is practical engineering. In today’s episode, we’re talking about crane failures.
This video is sponsored by Hello Fresh America’s number one meal Kit. More on that later.
Cranes are the backbone of just about every construction project.
All construction can be boiled down to material handling, taking delivery, storing, moving, and placing all the pieces and parts of a project. Of course, you can do a lot with your own sweat and muscles, and there are even tools to provide a mechanical advantage, allowing one person to lift a lot. But, anyone working in the trades will tell you there are plenty of jobs only a crane can accomplish. Heavy equipment amplifies the amount of work that can be done.
That’s so true with cranes that the question at most construction sites is not if a crane will be mobilised, but when and what type. Because they’re so pervasive and they do such a dangerous job of lifting massive objects high into the air. Occasionally, cranes fail. In this video, I want to walk through some of the reasons these failures occur, using historical accidents as case studies. I’ve got some demos set up in my garage with toy cranes, but these accidents are no joke. Crane collapses lead to property damage, injuries and fatalities every year, but they’re almost always preventable. So I’ll do my best to explain what could have been done differently for the cases described.
By the way, this is part one of a series on cranes. A future video will cover rigging and the things that can go wrong between the hook and the load. The first reason cranes fail is improper assembly or disassembly. Most cranes on construction sites are temporary. They’re not staying when the job is done. That means they either arrived under their own power called mobile crane, or they were shipped on trucks and assembled on-site, a process that can take days or weeks, depending on the size of the machine.
A crane can be extremely vulnerable during assembly or disassembly since all the components aren’t fully bolted together. A recent collapse in Seattle happened when a team disassembling a tower crane prematurely removed the pins holding sections of the tower together. Presumably, this was done to speed up disassembly. However, when winds picked up that day, major components of the crane were completely disconnected, being held together just by gravity and loose sliding connections between the members. It didn’t take long for the crane to collapse, killing four people and injuring many more.
In most cases, these cranes are expertly engineered for worst-case conditions. But the manufacturers are rarely the ones installing them on-site, so they provide detailed manuals for the crews assembling and disassembling them. Unfortunately, those manuals aren’t always followed to the letter. One of the worst crane disasters in modern history happened in 2008 in New York City. Crews were working to attach a tower crane to a building, when a major component of the attachment hardware, a heavy steel collar suddenly fell. As it fell, it crashed into the attachment points below, breaking them from the building and allowing the whole crane to overturn.
The cause of the accident was simple.
The crew assembling the crane didn’t follow the manual. Specifically, they didn’t attach that collar to the crane according to the manufacturer’s guidelines while it was bolted together and attached to the building. Seven people died as a result. Another collapse in Battersea, England, in 2006 also happened because the operator was using the wrong manual to assemble a crane. Instead of an eight-tonne counterweight, they used twelve tonnes, putting the crane way out of balance. Eventually, the bolts holding the slew ring failed, and the entire boom broke free, killing the operator and another person who was just fixing his car on the street nearby.
Once a crane is set up, the challenges in keeping it that way aren’t over. Many crane failures happen during everyday operations, and one of the biggest causes is overloading. Every crane has limitations in how much weight it can handle, but it’s not as simple as something like a bridge that has a single load limit. Not only can most cranes have a wide variety of different configurations, like different counterweights jib lengths and boom sizes. They also, by the very nature of their job, move. They slew, luff, telescope, traverse, boom up or down, et cetera. And more importantly, their load limitations depend on these movements.
Every crane has a tipping line.
That’s the line at which the machine will tip if overloaded. Any increase in weight outside the tipping line destabilises the crane if not balanced on the other side. The further away the load is from the tipping line, the greater the moment or torque on the crane. This is easy to demonstrate with my model. Using a spring scale, I can estimate the load required to tip the crane at different distances from the tipping line.
As the distance from the crane goes up, the force required to tip it over goes down proportionally. Cranes have a few tools to keep the load in balance. One is counterweights. These weights oppose the torque by balancing it on the opposite side of the crane. Another tool is outriggers. When there’s enough room to use them. These arms extend the tipping line, bringing it closer to the load and thus reducing the length of the lever. The crane can hold several hundred grammes with the outriggers extended, but it can barely even hold up the spring scale without them.
All those factors add up to a lot more than any operator can be expected to keep track of, which is why cranes have load charts. Reading these charts is pretty simple as long as you’re looking at the one that matches the configuration of your crane. Look at the furthest radius your hook will be from the centre line of the crane during the lift, and you’ll see the maximum allowable load the crane can handle. Of course, most modern cranes have sensors and electronics that can help an operator keep track of this on the fly.
Load moment indicators tell the crane operator when they’re getting close to the maximum. Many cranes will even lock out specific movements to prevent the crane from tipping or sustaining damage from overloading. That doesn’t mean it doesn’t happen, though. In 2016 in Manhattan, a crawler crane fell over as it was being laid down due to high winds. The boom and jib of this particular crane could be set down on the ground if things got too windy. But even with nothing on the hook, the load chart doesn’t allow the boom to be lowered below 75 degrees.
Unfortunately, the operator dropped the boom below this level and the crane fell, killing one person and injuring several others. Even if the crane can handle the load and do it stably, that weight doesn’t just stop at the base. It has to be transferred to the ground. And surprisingly, sometimes the ground can fail. The geotechnical engineers call this a vertical deformation, but you can just say the ground moved when it wasn’t supposed to. And this can happen in a couple of ways. The first is settlement. That’s when soil particles compress together when subjected to a load. It’s usually a slow process, but it can create issues at a construction site over time. Settlement can be solved in one of two ways. Sometimes compacting the subgrade before using it as a foundation is enough to make sure that it won’t compress further over time. For clay soils that are difficult to compact, it’s usually best to replace the top layer with something more stable, like crushed rock. The other type of vertical deformation is called a bearing capacity failure. In this case, the soil particles actually slide against each other in a shearing motion. These dowels represent soil particles, and you can see what happens if the Earth can’t withstand a vertical load. The particles below the base get forced downward while the adjacent particles bulge up on the sides.
Here’s an example using a tower crane.
With the hook at the end of the boom, the crane can hold 150 grammes without tipping on a stable surface. But when set on top of loose sand, things aren’t quite so static. The soil isn’t able to support the 2ft in compression. Instead, it gives way, allowing the crane to topple.
In 2012, this crane fell over while lifting a part of a ship in Vietnam. The cause is obvious: the ground wasn’t strong enough to withstand the load. This accident killed five people. Geotechnical engineers can estimate the bearing capacity using simple tests, so there’s no good reason this should ever happen. If the ground can’t handle what’s required for the crane, the solution is simple. Distribute the load over a larger area. This is often done using steel plates or wooden constructions called crane mats.
Water also affects bearing capacity.
The strength of the soil is primarily a function of friction between soil particles. If water gets into the space between those particles, it pushes them away from each other, reducing the friction and weakening the soil. If you’ve ever stepped in the mud, you have some intuition about this.
In 2013, a massive crane helping with the construction on the Brazil World Cup Stadium collapsed while lifting a roof section into place. The cause of the collapse was a bearing capacity failure of the soil beneath the crane exacerbated by several days of heavy rainfall. So, keeping the site well-drained from water is essential, and again, replacing crummy soil with a stable free-draining material is usually best practice.
The final cause of crane collapses that I want to discuss is wind, or rather, neglecting the power of the wind to overload. In 2019, a severe thunderstorm led to the collapse of a tower crane in Dallas, killing one person and injuring several others. The fault for the accident is still being litigated, but it brings up one thing many don’t realise. Most tower cranes are designed to withstand very high winds, but only under certain conditions. When winds are high, operators have to disengage the clutch, so the crane can freely point into the wind. This is called weather veining. If the boom is locked, it can end up broadside to the wind, significantly increasing the forces on the crane.
In the video of the 2019 collapse, you can see two cranes pointing in different directions and only one of them failed. That suggests that an operator may have forgotten to secure the crane properly or the crane malfunctioned and couldn’t weather vein into the wind. In September 2017, three cranes at separate construction sites in Florida all collapsed on the same day due to winds from Hurricane Irma.
All three cranes were the same make and model, and they were all secured to weather vane as required. Luckily, no one was injured at any of the sites since they were shut down due to weather. The winds during the storm were more than 125 mph or 200 kilometres per hour. In some places, that’s far more than the maximum design wind speed for the cranes, which was 95 mph or about 150 kilometres per hour. As the saying goes, there’s always a bigger storm, and in this case, Hurricane Irma just delivered winds that were well above the requirements and codes that cranes are required to meet.
However, since only the single type of crane failed during the storm, investigators recommended some changes to the design that may make them safer in the future. Situations like what happened in Florida are the exception, not the rule, though. As I mentioned, nearly every crane accident is easily preventable. In 1999, one of the largest cranes in the world collapsed during the construction of Miller Park in Milwaukee, now called American Family Field. The crane was lifting a 500-tonne roof section of the Stadium at 97% of its rated capacity, but it wasn’t just the weight of the structural members bearing on the hook.
Steel assemblies can appear pretty slim, but that doesn’t mean they can’t catch the wind. Watch what happens when I put my leaf blower on this steel truss. It’s a little hard to see the spring scale, but a little bit of wind goes a long way. By my estimation, the wind is adding about 15% to the load on the hook. It’s more than the margin of error when you’re operating at maximum capacity, and that doesn’t even consider the huge horizontal loads affecting the crane. These machines are rarely capable of withstanding much force in any direction other than straight down, so their load charts generally disallow operation when winds are high.
On that fateful day in 1999, the crane operator neglected to include the additional force from wind loading when assessing the Crane’s capacity, and so it ended up overloaded. A safety Inspector happened to catch the entire collapse. On camera, three construction workers were killed during this incident, and a sculpture of them still stands at the Stadium in their memory.
As you may have noticed, there are technical reasons that cranes fall over, but there are also underlying human factors as well. That’s why it’s best practice to create a lift plan anytime a crane is used. That involves taking the opportunity before the load is in the air to consider all aspects of the lift and what could go wrong: weight, dimension, the centre of gravity, and lifting points of the load, the path that will travel during the lift, the capabilities of the crane, outside factors like wind and communications during the whole process. For complicated lifts, these plans can take days or weeks to prepare with detailed engineering reviews to ensure that nothing goes wrong once the load is on the hook.